Keeping Quantum Information Safe: Protecting Qubits from Noise
- Quantum Valley
- Nov 16, 2024
- 3 min read
Updated: Nov 17, 2024
Research and post by Student - Flora Dixit
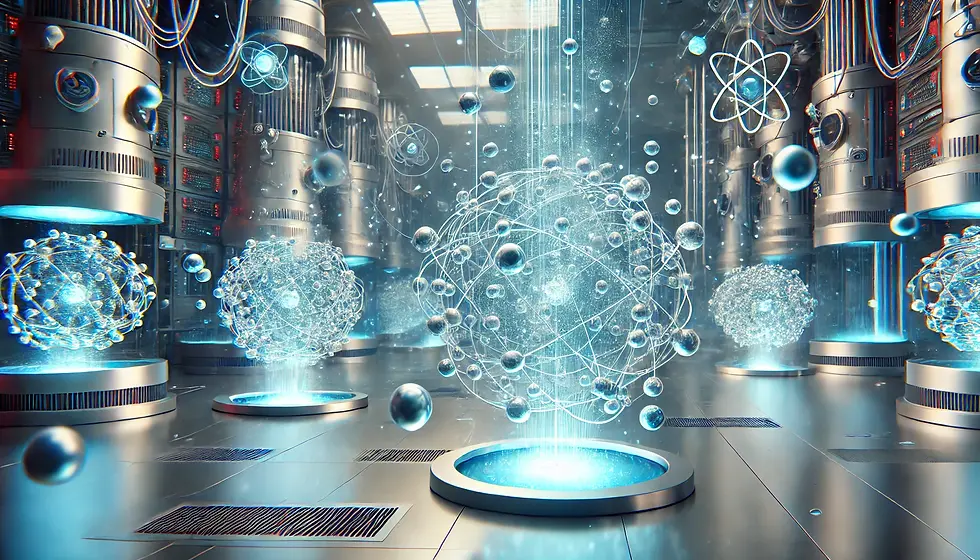
Imagine a computer so powerful that it could solve problems in minutes that would take classical computers billions of years. That’s the promise of quantum computing. However, there’s a catch – qubits, the building blocks of quantum computers, are extremely fragile. They’re susceptible to noise from the environment, which can corrupt the information they store and lead to errors in computations.
This blog post will explore how researchers are tackling this challenge and working to protect qubits from noise, making quantum computing more robust.
The Challenge of Noise
Qubits are incredibly sensitive to their surroundings. Even the slightest changes in temperature or interactions with nearby molecules can cause them to lose their quantum information. This process, known as decoherence, is a major obstacle to building practical quantum computers [1].
Quantum Error Correction to the Rescue
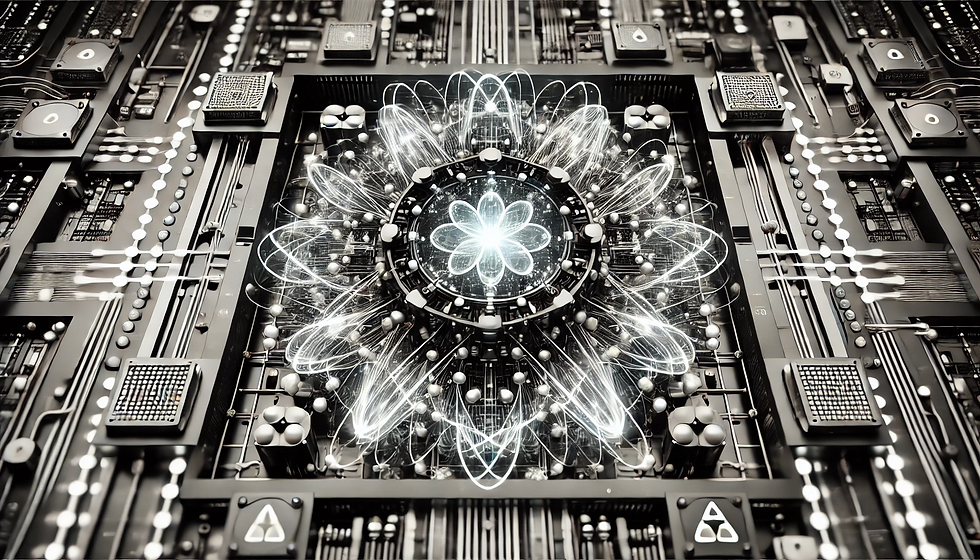
One of the most promising approaches to address this challenge is quantum error correction (QEC). Think of it as a shield for quantum information. QEC techniques involve encoding the quantum information across multiple physical qubits. By spreading the information out, QEC codes can detect and correct errors that occur on individual qubits.
Several types of QEC codes have been developed, each with its own strengths and weaknesses. Some popular ones include:
Shor code: One of the first QEC codes, it can correct both bit-flip and phase-flip errors, two common types of errors that can occur in qubits [2].
Surface code: This code uses a 2D grid of qubits and is known for its high tolerance to errors, making it suitable for large-scale quantum computers [3].
Fault-Tolerant Quantum Computing
Another important concept in protecting qubits is fault tolerance, which is the ability of a quantum computer to continue operating correctly even when some of its components, like qubits or gates, fail. Achieving fault tolerance is crucial for building reliable quantum computers that can tackle complex computations. In addition to quantum error correction codes, researchers are exploring various fault-tolerant architectures and approaches. One such approach is the concatenated code method, which involves nesting smaller quantum error correction codes within larger codes, providing layers of protection against errors [4]. Another promising avenue is bosonic codes, which use continuous variables rather than discrete qubit states, offering a new way to protect information from common types of noise [5]. These approaches, combined with advanced error correction, contribute significantly to the robustness of quantum computers. However, researchers are also investigating unique particle states for even more durable qubit structures.
Majorana Fermions: A New Hope?
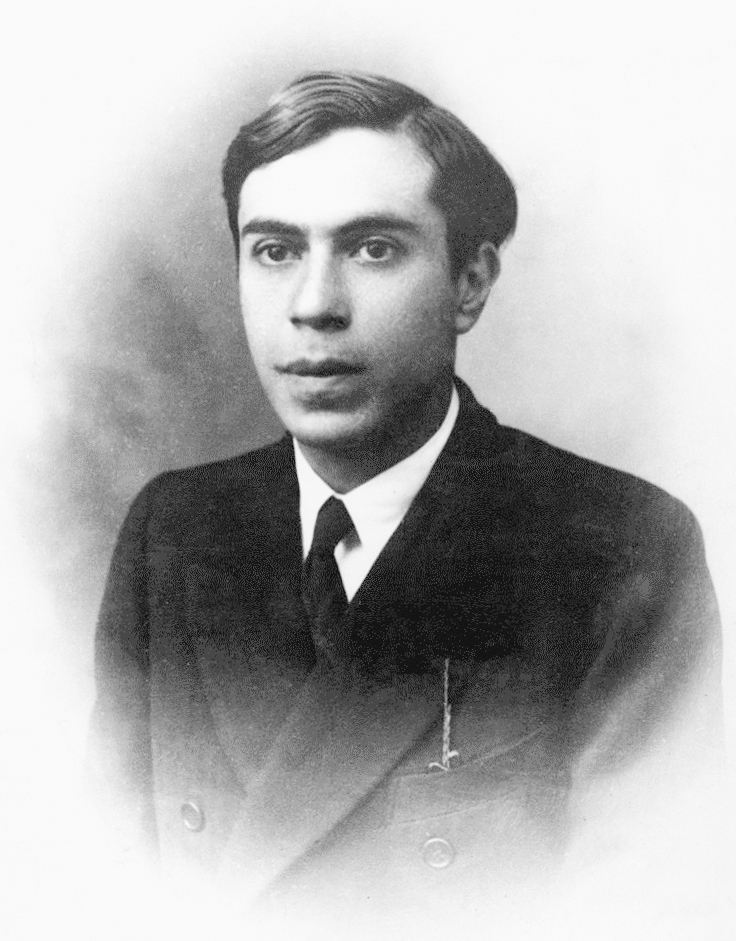
One exciting area of research in fault-tolerant quantum computing involves Majorana fermions. These particles are unique because they are their own antiparticles, meaning that a Majorana fermion is identical to its own antimatter counterpart [6].
Alexei Kitaev proposed a method for creating topological qubits using Majorana fermions. These topological qubits would be inherently protected from local errors due to the unique properties of Majorana fermions [7]. Kitaev’s research suggests that Majorana fermions could be found at the ends of special 1D quantum wires. If realized, this could lead to the development of more robust and fault-tolerant quantum computers.
Conclusion
Protecting qubits from noise is a major challenge in the development of quantum computers. Quantum error correction, fault-tolerant quantum computing, and alternative methods such as concatenated and bosonic codes are all crucial approaches to overcoming this hurdle. Additionally, the development of topological qubits using Majorana fermions is a promising area of research that could lead to more robust and reliable quantum computers in the future.
References
Quantum Computing Challenges. The Quantum Insider. Retrieved from https://thequantuminsider.com/2023/03/24/quantum-computing-challenges/.
Quantum Error Correction. Microsoft Azure Quantum Documentation. Retrieved from https://learn.microsoft.com/en-us/azure/quantum/concepts-error-correction.
Krinner, S., Lacroix, N., Remm, A., et al. (2022). Realizing repeated quantum error correction in a distance-three surface code. Nature, 605, 669-674. Retrieved from https://www.nature.com/articles/s41586-022-05434-1.
Knill, E., Laflamme, R., & Zurek, W. H. Concatenated Quantum Error-Correcting Codes. Retrieved from https://arxiv.org/html/2411.03202v1.
Noh, K., & Chamberland, C. (2023). Fault-tolerant bosonic quantum error correction with the surface–Gottesman-Kitaev-Preskill code. Progress in Quantum Electronics, 92, 100365. Retrieved from https://www.sciencedirect.com/science/article/abs/pii/S0079672723000459.
Physicists uncover new behavior of quantum superconductors. Phys.org. Retrieved from https://phys.org/news/2024-10-physicists-uncover-behavior-quantum-superconductors.html.
Kitaev, A. Y. (2013). Fault-tolerant quantum computation by anyons. Annals of Physics, 303, 2-30. Retrieved from https://arxiv.org/abs/1312.6583.
About Author
Flora Dixit is a pioneering quantum computing student and STEM advocate. She studied Quantum Information Sciences and Engineering at COSMOS, exploring quantum computing's potential to revolutionize various industries. As president of her school's Girls in Tek club, she empowers young women to pursue STEM careers through workshops, mentorship, and networking events. Flora's expertise in quantum computing, AI, and CS, combined with her passion for inclusivity, positions her as a future tech leader ready to tackle the challenges of the digital age. In addition to her academic pursuits, Flora is a dedicated TaeKwando practitioner and has proudly represented Team USA, winning gold at the International World Championships